Earth life is extremely resilient, and has been for most of its history. One reason is its inherent ability to adapt to changing conditions and take advantage of varied, even extreme environments. In doing so, it also modifies and creates new environments, eventually transforming the surface of the whole planet. Planet Earth today is very different from the planet on which life emergedâprecisely because life emerged on it.
Moreover, Earth has been harshly inhospitable to the emergence of new forms of life for billions of years, courtesy of current Earth life and the highly oxidizing atmosphere it has created. Life is truly a planetary phenomenon, as my colleague Andrew Knoll showed convincingly: life and planet evolved together, dynamic and inexorably linked.
17
Planets may be good places to sustain life, but we have not yet answered the question, Is Earth the ideal place for life to emerge?
CHAPTER TEN
PLACES WE COULD CALL HOME
A
long time ago, in a galaxy a lot like ours, a star formed. Soon several planets formed too. Three of the planets were close to the star. They weren't very big, and they were almost entirely rocky.
The smallest and the coldest of the three had an atmosphere of carbon dioxide and sulfur dioxide produced by volcanoes. They acted like a greenhouse that kept its surface warm, and so some of the surface water was able to liquefy. Together, the atmosphere and the oceans made the chemistry on the planet's surface varied and funâand gave life a good chance to emerge. The party ended after a few hundred million years, in geological terms nothing more than a summer vacation in Alaska. The planet, being small, had a hard time holding on to its atmosphere and supporting
enough geological activity to replenish it. It just could not keep warm, inside or out.
The other two planets, however, were larger, so they had more water and a more substantial atmosphere, though they too were all rock at heart. One of them, the planet closer to the star of the pair, wasâlike its smaller cousinâquick to change, although in an opposite sense. Its ocean began to evaporate under the star's glare, and the addition of the water to the atmosphereâwhere it served as an excellent greenhouse gasâprompted a runaway loss of the rest of the water, leaving nothing but a rocky desert behind.
1
On both planets, however varied the chemistry might have been initially, the geochemistry became increasingly limited, and gradually settled into an inactive equilibrium. The future development of these planets soon became predictable because it was very simpleâdescribed by the basic laws of physics and chemistry. They joined most of the rest of the galaxyâfrom gas giants to stellar remnantsâin a slow, dull process of cooling with no further chemical changes, simply whirling around under the influence of gravity.
The third planet was luckyâlarge enough and warm enough to replenish and keep an atmosphere and to recycle its surface material, yet not too hot, so that it could keep most of its surface water liquid. The chemistry on this planet was fun and varied too, but even more so than on its little cousin. An existing geochemical cycle of carbon dioxide soon took the direction of planetary development farther and farther away from its geochemical equilibrium. Something fundamentally different was happening. About 2 billion years into
this, the atmosphere and the oceans became increasingly richer in the very reactive free oxygen. Real fun for a chemist!
While dull equilibrium ever more firmly gripped the first two planets, the third was becoming even wilder, reacting in complex ways to outside disturbances, such as the asteroid impacts that for a time incessantly bombarded the planet's surface. This went on for about 4 billion years, although the asteroid impacts eventually petered outâuntil finally this complex chemistry harnessed enough energy locally to eject small pieces of the surface (and itself!) into orbit, eventually to its moon and back, eventually away from the star and into the galaxy.
From the perspective just described, the fate of three planets as viewed from above, from the vantage point of the distant stars, might appear very natural. Just as stars are different, yet any star is much simpler than a planet, planets are different too and some develop a complex chemistry. Life as viewed from the stars would be indistinguishable from any other kind of chemical process, except for the complexity of its outputs. Much as we might speak of a water planet, because its chemistry outputs great oceans, we might, in a galactic sense, speak of a life planet because that's what, given its constituents and its place in a solar system, its chemistry leads to. And just like liquid oceans might be only a part of the history of a type of planet, so it could be with life.
You probably won't be surprised to learn that the three planets I've been describing are planets we know wellâMars, Venus, and Earth. In this context, Earth seems like the place to be. But is Earth the ideal planet for life? I come back to the
question I posed in the beginning of the book. Now I'll try to answer it. And the answer is no. Super-Earths are better.
For any planet we find, super-Earth or not, we try to assess habitability. It is a tricky concept. For example, if we define as habitable any place we humans can inhabit without special protection, there will be many places on Earth that fail to qualify. If we allow for areas where we could survive given our best technology, the equatorial regions of Mars would qualify but not a fiery furnace like Venus. Some Earth microbes are so hardy they could survive in the Martian soil. Clearly the habitability of a planet depends on its location: far from the Sun it is too cold, much closer to the Sun is too hot. This range of comfortable distance is called a habitable zone, and is indicated by the presence of surface water.
2
Every star has one.
I am looking for environments that make complex molecular chemistry viable. I am interested in pathways to (origins of) life in the Universe, so I am looking for planets that maintain surface temperatures in which large molecules can survive and can attain chemical concentrations and can be sufficiently stable over time. Although the habitable zone concept is helpful in terms of temperature, it is not sufficient. There are many other factors that contribute to the habitability of a planet. Given that our knowledge of the conditions on super-Earths will be very limited until we actually visit them one day in the future, I prefer to talk about their
habitable potential
.
What is the habitable potential of the first known super-Earths? Let's visit some of them. Gliese 876d orbits a small cool M dwarf star once every two days! That means that its orbit
is only 2 percent of the Earth-Sun distance (or 0.02 AU). Its close proximity means that, although its star is only a third of the weight and size of the Sun and only half as hot, the surface temperature on Gliese 876d must exceed that of sweltering Venus (about 730 K). Super-Earth Gliese 876d is not in the habitable zone and has no habitable potential (
Figure 10.1
).
There are two other, Jupiter-like, planets in this planetary system.
3
They were discovered in 2000 by Geoff Marcy and the California-Carnegie team and have the designations Gliese 876b and 876c. They have masses equal to about 2 and 0.6 Jupiters, respectively. Despite being inside the habitable zone, these planets are gas giants. Lacking any solid surface, they have no habitable potential. However, either of them might have large moons that could have excellent habitable potential. Unfortunately, these two Jupiter-like planets have close orbits and interact gravitationally with each other, so it is likely that they could not have and retain large moons.
Another M dwarf star, Gliese 581, is known to have three super-Earths (
Figure 10.1
). A hot Neptune of 25 M
E
(called Gliese 581b) has been known since 2005; it orbits the star with a period of 5.4 days. Later, Michel Mayor's Geneva team found two smaller planets that could be the closest resemblance to Earth yet, as they seem to be barely inside the habitable zone. Because Gliese 581 and 876 are very similar stars, it is easy to compare their planetary systems and habitable zones. The simple assumption that super-Earths ought to have atmospheres leads to the habitable zone shown in the figure, derived from the work of Franck Selsis and colleagues.
4
The super-Earth with the best habitable potential is the planet Gliese
581d, which has a mass of eight Earths and is farther from the star; its atmosphere helps keep the planet warm. Gliese 581c, which has a mass of five Earths, is likely too hot, and there is an even hotter super-Earth E inside the orbit of B.
Â
FIGURE 10.1
.
Two planetary systems and their habitable zones. The super-Earth in Gliese 876 is the inner D planet. In Gliese 581 , the inner B planet is actually a hot Neptune. Planet Gliese 581d is the only super-Earth that happens to orbit within a habitable zone, barely; it has a slightly eccentric orbit. Gliese 581 e, a fourth planet not shown here, is barely two Earths but orbits the star inside the orbit of planet B and is too hot.
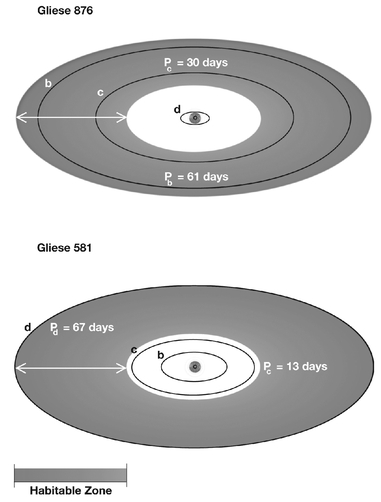
The planetary system Gliese 581 is interesting in another way: most, if not all, of the planets must have formed farther from the star than they currently are. First, there is not enough material in a proto-planetary disk for such a bulky planet as the hot Neptune, Gliese 581b, to have formed just a dozen stellar sizes away from the star. Second, the temperature in the disk is too high for the large planet to have accumulated. It is much more likely that the hot Neptune, just like the hot Jupiters described earlier, formed farther out and was pushed close to the star by the disk. If that's true, then the two super-Earths must have formed even farther out in the disk. They could be ocean planets, as they must have formed outside the disk's snow line, where water freezes and accumulates easily. Some of this surface water would have liquefied when the planets were pushed closer in. However, they could be gas-rich planets, similar to the recently discovered planets GJ 1214b by David Charbonneau's MEarth Project, and the planets in the Kepler-11 system. These are all of similar massesâa few Earths, and they are all of very low density that requires them to be mini versions of Uranus and Neptune.
Two factors make super-Earth planets more habitable than a planet the size of our own. First, by being more massive these planets have an easier time keeping their atmospheres and water from evaporating. This is very important for the ones that would orbit closer to their stars than, say, Mars is to
the Sun. Second, if they are rocky super-Earths, their tectonic plate activity is as high as the Earth's or even higher, according to our theoretical models.
5
This is important for life and its origins. In our Solar System, Mars never had moving plates, and Venus seems marginally capable of moving its plates. It seems that our Earth barely made it!
Tectonic plate activity is what we observe as continental drift on Earth. Modern GPS technology allows us to measure this motion. But why is it beneficial to life? The answer, in a nutshell, is stability and chemical concentration.
6
Over billions of years the Earth has kept its surface temperature stable. The oceans, for example, have generally always been liquid. We know this from geological evidence. We also know that our Sun has brightened up by 30 percent since the Earth formed. The solution to this seeming mystery appears to be a global geochemical cycle. The Earth is a large ball that is very hot and boiling inside. On the surface this energy moves the set of rocky continental and ocean plates around. The essential thing about planetary plate tectonics is
exchange
. The molten, mixed interior can exchange chemical elements with the surface and atmosphere and vice versa. The elements are not simply recycled in this process, but their chemical transformations and concentrations exchange energy in a rich dynamic equilibrium. The alternative is a much poorer steady-state equilibrium that will set in on the inside and at the surface with no local energy sources. Thus plate tectonics makes a planet dynamic, renewing, and vital. As Ward and Brownlee put it, plate tectonics promotes environmental complexity.
7
The exchange occurs through global cycles.
Â
FIGURE 10.2
.
The CO cycle (a.k.a. carbonate silicate cycle) starts with the volcanoes on the left that provide the supply of CO
2
gas, which gets absorbed by water in the atmosphere, rains down, erodes the continents, and ends up recycled back into the hot interior via plate tectonics, only to return again.