Life as we know it
The maximum depth of the Burgess Shale environment was 70 metres, and that is a sunlit environment where colour and ornaments are in operation today.
Hallucigenia
was a Burgess velvet worm and
Wiwaxia
a form of Burgess bristle worm, and both bore large, fearsome spines.
These animals lived on the sea floor and their spines were directed upwards into the water. They were aimed at predators swimming above them - they were armaments
and
ornaments (it is generally thought that bristle worms evolved spines in response to predators . . . predators with eyes, that is). The velvet worm
Asheaia
did not evolve spines, but rather formed an association with a sponge. Like comparable animals today, it probably evolved precisely the same colour as the sponge, or even stole the sponge's pigment. It is not easy to pick out a bristle star or crustacean living on a sponge today since their camouflage is perfect. Today's adaptations to visual predators probably evolved quickly during the Cambrian. From the beginning of the Cambrian up to today, the world has been adapted to predators with vision. For that same basic concept to have remained in place, unaltered, over some 540 million years illustrates just how powerful it really is. Powerful and stabilising.
The iridescence of
Wiwaxia
,
Canadia
and
Marrella
from the Burgess Shale probably functioned to deter predators. Like
Hallucigenia
and
Asheaia
, these animals could be considered slow-moving chunks of protein, but their protective spines would have appeared as iridescent, changing colours as predators approached. Changing colours or flashing lights are more conspicuous than a steady light, and so the visual warning created by the shape of the spines would have been enhanced. As explained earlier, both the shape and colour of the spines are adaptations to the vision of their predators - they are ornaments.
Using only their eyes, South American side-necked turtles can quickly estimate the nutritional value of potential prey in their environment. They will, however, only attack those animals that are vulnerable, regardless of their nutritional content. So a turtle will ignore a highly nutritional animal that is difficult to capture in favour of slow, easy pickings.
Wiwaxia
would have reflected all the wavelengths present in the Burgess Shale environment, and at least some of those wavelengths would have been used by its predators to achieve vision, so the iridescence would have been seen. It signalled that
Wiwaxia
was no easy picking. Basically, light display and vision would have been as important in Cambrian environments as they are in environments at comparable depths today.
I have spoken of the Cambrian as a transitional period, but it seems that the turmoil really was confined to those five million years or less at the beginning of the Cambrian - the Cambrian explosion. After this, after everything had adapted to vision, stability began to replace the chaos. The arthropods
Isoxys
and
Waptia
are genera well known from Burgess Shale fossils, 515 million years old. But they were discovered also in the Chengjiang site of China, 525 million years old. So those genera existed for a considerable period of time - at least ten million years.
Anomalocaris
is known from an even longer stretch of the Cambrian, and the list continues, with life appearing quite well conserved following the Cambrian explosion. Vision entered the Earth with a bang, or a flick of the light switch, and then things settled down. The introduction of vision triggered a scramble to occupy the new niches that had opened up as a consequence. Once all those niches had been filled, micro-evolution resumed. Think of the dinosaurs. Dinosaurs occupied the top predatory niches and kept mammals low down in the food pyramid. Mammals could only take over once the dinosaurs were removed - when niches are filled there is stability in the system, a stability which resists change.
Why vision and not other senses?
Light is only one of a suite of stimuli in today's environments. Maybe the other stimuli should be considered in relation to the Cambrian explosion in much the same way as light has been considered in this book. Could the light switch really be the sensory switch, where all senses were introduced on Earth at the dawn of the Cambrian explosion? Or did the other major senses of animals today evolve gradually before and after the Cambrian explosion - were they subject to micro-evolution rather than macro-evolution?
First of all, what are the senses other than vision? A sense is the ability to detect and be conscious of the outside world. A sense involves a stimulus and a detector. Excluding eyes and vision now, detectors are usually one of two types - chemical or mechanical. Magnetic detectors also exist, which track the direction of the Earth's magnetic field. The
magnetic sense is best known in insects and chordates, such as the homing pigeon, which can determine its geographical position by using a âmagnetic map'. Some fishes, notably sharks, use the magnetic sense to hunt prey, but usually this sense is employed for orientation purposes. It is not known to have played a role in predator-prey relationships until after the Cambrian, and so can be excluded as a possible cause of the Cambrian explosion.
A case of micro-evolution
Chemical detectors detect chemicals and give rise to the senses of taste or smell. They contain nerve fibres that produce electrical impulses when contacted by specific chemicals. In its most rudimentary form, a nerve fibre terminates at the outer surface of the host animal and is free to be stimulated by chemicals that contact the animal. This can become more complex in different ways. Increase the density of the exposed nerve fibres and the animal becomes more sensitive to the chemical detected. Mix these nerves with different nerves sensitive to other chemicals and the animal becomes receptive to an array of chemicals. Then hold the nerve fibres away from the surface of the animal, so they protrude into the environment, and the animal becomes increasingly sensitive to chemicals. This is because the sticky boundary layer that surrounds animals acts less as a barrier to chemicals. Also, at a distance from the body surface there is less ânoise' or background chemicals coming from the host animal itself. Nerves that enter the environment can be protected within hairs. The detectors can range from a single nerve fibre within a single-pore hair to bundles of nerve fibres inside a hair with many perforations. And increasing the density of such hairs on the animal can increase the complexity and sensitivity of the hair system further. But the same pattern emerges however sensitivity is increased. This is a pattern that prevents chemical detectors from causing an explosion in evolution.
For every complex, highly sensitive detector of chemicals there is a well-defined and gradual evolutionary path. A detector in the form of a clump of hairs would have evolved from a few hairs, which in turn would have evolved from a single hair. That single hair would have
evolved from a single bump in the outer surface of the animal, which would have derived from a nerve penetrating the flat surface of a previous ancestor. But importantly, this path is rather smooth. In other words, the evolution of smell and taste over geological time was linear - it involved a series of numerous but gradual transitional stages. The evolutionary ride was also smooth.
Perhaps âlinear' and âsmooth' are exaggerations. Hairs, for instance, involve hard parts, and, as we know, hard parts did suddenly emerge overnight on the geological timescale. So at this point in history, the sensitivity of smell and taste detection may have jumped up a notch. But that jump would not have been monumental because the information supplied by the nerve fibres did not suddenly increase
several
times. And this particular jump was probably the biggest of all for chemical detectors, but it happened
during
the Cambrian explosion, and so could not have been the trigger. The evolutionary ride for smell and taste, nonetheless, would have been littered with modest bumps.
Mechanical receptors are so called because they sense physical movement in the environment. They contain nerve fibres that produce electrical impulses when they are themselves moved. This happens as a consequence of contacting an object or movement in the surrounding water or air. Mechanical receptors are responsible for the senses of touch, hearing/vibration detection, and gravity, temperature and pressure sensitivity. Like chemical detectors, mechanical receptors occur in a variety of shapes and sizes, but they also demonstrate that same evolutionary pattern. In theory, the evolution of mechanical receptors took place in small steps.
The evolution through geological time of chemical and mechanical receptors cannot be compared with the evolution of light detection. There is no event in the evolution of receptors of other senses that can match, or even come close to, the evolution of the lens. Chemical and mechanical detectors certainly would have become more efficient throughout the Cambrian explosion, but not to the extent that they would have changed the entire behavioural system of animals. There is no case of a receptor suddenly changing in efficiency âa hundredfold', like the change from a light-sensitive patch to an eye capable of producing visual images. Here lies the fundamental difference between
light detectors and the receptors of other stimuli - those of other stimuli still work at their intermediate stages of complexity and efficiency. The evolution of receptors for stimuli other than vision can theoretically show a linear progression, but a light perceiver with an inadequate lens has little advantage over one with no lens. The theoretical intermediate stages of a lens increase light perception only slightly, but when a complete, fully focusing lens is formed, the increase suddenly becomes vast. This leap in efficiency is so glaring that it led Darwin to single out the eye as the thorn in the side of evolutionary theory. But it also indicates that if the evolution of one type of receptor could trigger the Cambrian explosion, that receptor would be an eye. The evolution of the eye from a rudimentary light perceptor - that single jump from ânot to see' to âsee' as described in Chapter 7- was a small step for anatomy but a huge step for animal behaviour.
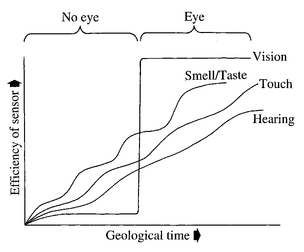
Figure 9.3
Graph showing the very approximate evolution of receptors for different stimuli throughout geological time. Vision is the only sense that can divide geological time into two distinct phases.
A presumed Precambrian history of electrical wiring and information processing
There is the evidence to be gained from the history of the nervous system and brain capacity to process the information collected by the detector. We now know that the eye
did
suddenly appear at the beginning of the Cambrian, and that it instantly became a common characteristic. A considerable number of Cambrian arthropods possessed eyes, so they must have worked then as they work today. For an eye to work, sizeable brain and nerve cables are required, and these were in part borrowed from other senses. This is the most conceivable way in which an eye can suddenly achieve vision, after its leap from simple progenitors, the light perceivers. What does this borrowing tell us? It indicates that at least some senses had evolved to a reasonable degree of sophistication before the Cambrian, so that they had established a nerve network including brain space. In turn this means they could not have triggered the Cambrian explosion, which leads us on to another interesting detail in the evolution of senses other than vision, a detail that emerges when the different animal phyla are compared.
Evidence from evolutionary history
Up to now we have been interested in the evolution of senses throughout geological time, but we should forget about time for a moment and look again at the evolutionary tree of multicelled animals, the tree that shows the order of genetic mutations and establishment of internal body plans. This will reveal the evolution of sensory systems throughout the evolution of phyla, rather than time. And generally we find that the first phyla to branch off the tree are represented by species today with simple nervous systems.
Mechanical reception, specifically touch, occurs in sponges by general cellular irritability. In the more evolutionary derived phylum, the flatworms, it occurs by the stimulation of free nerve endings. Even more derived phyla contain more sensitive mechanical receptors, which can detect pressure waves created by the low-frequency vibrations of
distant objects. The most derived phyla can even detect sound waves or high-frequency vibrations by means of special phonoreceptors.
Other senses show a similar pattern of gradual development throughout evolution. Sponges are without specialised chemical receptors, but again taste/smell is a general property of the body surface. Sponges respond to chemical irritants by contraction of the opening to the body cavity, which restricts the flow of water and the irritant through the sponge. But it is the contractile cells surrounding the opening that respond directly to the irritants - no specialised receptors are involved. The next phylum to branch off the evolutionary tree includes sea anemones and jellyfish. These animals possess simple chemical receptors in their mouths and tentacles, which can distinguish between different food types - anemones and jellyfish prefer some foods to others. The chemical senses are even better developed in molluscs, echinoderms (starfish phylum), arthropods and other more highly derived phyla, where they are also used to locate food sources.