The image-forming structure in the âpinhole eye' of nautilus is a small pupil, or âpinhole', formed in its dark iris. Light is not focused, but is received only through the pinhole, providing at least some degree of control. To gain accurate directional information, the retinal mosaic is remarkably fine so that light coming from a single point will illuminate several receptor cells. But serious disadvantages are inherent with
this type of eye, which accounts for its rarity. A bright image requires a large pupil, whereas a sharp image requires a small pupil. The nautilus' solution - a large range of pupil sizes or pinholes - unfortunately results in blurred images.
In his book
Optiks
, published in 1704, Sir Isaac Newton revealed his plans for a telescope without a lens but with a curved, concave mirror instead. This mirror would focus light towards a focal point, in the same way that a modern satellite dish focuses radiation towards its receiver. At the focal point was positioned a small, flat mirror, angled to redirect the focused light out through a gap in the side of the telescope - the eyepiece. This âNewtonian' telescope works well - it is popular today.
Curved mirrors can also be successful substitutes for lenses in eyes. The scallop has many eyes just inside the edge of its shell. These eyes appear silver, like tiny mirrors - and indeed they do contain mirrors. Within each eye, a hemispherical concave mirror similar to the reflector in a car headlight lies
behind
the image-forming retina. Light passes almost unfocused
through
the transparent retina before it is reflected back, this time focused by the mirror. The light is focused precisely at the position of the retina. And now the retina absorbs the light rays, and an image is grabbed. The mirror is achieved by the same mechanism found in the skin of the Mexican cave fish - stacks of thin layers of various thicknesses. The mirror eye is an improvement on the pinhole eye because it can focus light. But with light first passing through the retina unfocused there is potential for
this
to be detected, and so the performance of the eye is limited. For this reason, the mirror eye is confined mainly to the scallop and a few related clams.
The third type of simple eye in molluscs is found in a snail. The snail has an eye separated from the skin and containing a large spherical lens. This eye is known as the camera-type. It works in the same way as a camera in that a single lens focuses light on to a film, or retina, with an adjustable iris included to alter the quantity of light passing through its âpupil'. The general design is quite simple, but it is ideal for seeing images, and the variety of camera-type eyes to be found in other animal phyla testifies to its success.
The most efficient eyes of bristle worms belong to a group known as
alciopids. One member of this group lives on the surface of the sea and possesses camera-type eyes complete with a paired retina with refined layering, two distinct layers of âhumoral' material which fills the eyeball, and a well-developed spherical lens and âcornea' - the outer covering of the eye. The retina contains about 10,000 light detection cells, and is positioned at the focal plane of the lens - the position where the lens focuses an image.
Figure 7.2
The three types of simple eye - pinhole, mirror and camera-type - and their effect on light rays. Light receptors (retinas) are shaded. The mirror eye has an underlying mirror (dashed region) and the camera-type eye has a lens, both of which focus light to form clear images.
The camera-type eye is the standard hardware for vision in vertebrates, both on land and underwater. Humans are one beneficiary, but in addition to bristle worms and molluscs it has also emerged in spiders and crustaceans within the arthropod phylum, velvet worms within a phylum all of their own, and in box jellyfish within the cnidarian phylum. The precise design of the camera-type eye is determined by how the lens is formed - it can be formed either inside the eye, or outside, where it is actually part of the skin or exoskeleton, and is technically the cornea.
Focusing is all about bending light rays from different parts of the environment towards a common point. There are two factors which affect the bending of light rays - the differences in materials either side of a boundary, and the angle of that boundary relative to a light ray (think of a prism). Adaptations to vision on land are different from
those underwater because, as we learnt in Chapter 3, light behaves differently in air compared to water - there is a material difference. Light does behave similarly in water and in the cornea, so it barely recognises a boundary as it enters the eyes of aquatic species. In this case, the lens within the eye must be responsible for most of the focusing. But light recognises a considerable difference between the cornea and air, and it is bent as it crosses their boundary at an angle. So the cornea of land animals acts as a powerful lens in its own right.
James Clerk Maxwell tackled the subject of underwater focusing in the nineteenth century while contemplating his breakfast herring. Following a spontaneous dissection, Maxwell noticed his herring had a spherical lens. This is typical in fish - it bends light rays more than a thinner, oval lens because its surfaces are more steeply curved and present steeper tangents to light. But there is a problem with a spherical lens - spherical aberration. This is the reason why we do not use spherical lenses in cameras, and instead choose a series of âoval' lenses. Spherical aberration occurs when light striking the periphery of a lens is focused at a different plane to light striking the central axis of the lens - the peripheries bend light too much. So to focus both sets of rays simultaneously, the retina must be in two places at the same time. This is impossible. But fishes
can
see by focusing very sharp images - the question is âHow?' There is only one solution here, and Maxwell worked it out.
If the curves, or rather tangents, are made less steep by flattening the lens, the focal point moves too far from the lens - a huge eyeball would be needed to house the retina. So if the angles can't be changed there is only one other option for solving spherical aberration - change the materials. And indeed Maxwell suggested that the material of the fish lens is not uniform but is graded from the centre outwards.
Today we know from precise measurements that the periphery of the fish lens has optical properties similar to those of water and causes light to bend only slightly. This compensates for the comparatively glancing angle with which light strikes the edge of the lens, which alone causes considerable bending of the light path. Near the central axis, this angle is not glancing but nearer to 90°. So to keep central light rays synchronised with those from the periphery of the lens, the lens material in
the centre is optically very different from water and causes light to bend more, but also to slow down more. This effect on the speed of light is important since the path through the centre of the lens is now the shortest. To sum up the effect of this lens,
all
the light rays striking the eye at one instant will be focused on to the same point on the retina at the same time. Clever! Now a sharp image is formed . . . and formed equally well in all directions.
Not surprisingly, the graded lens has been an evolutionary success in the water - it is also found in marine mammals, tadpoles, and some molluscs such as octopuses, squids, cuttlefishes, winkles, conches and pond snails. Michael Land, an authority on the eyes of living animals based at Sussex University in England, calculated that if nautilus had a camera-type eye of the same size as its pinhole eye, it would be four hundred times more sensitive and have one hundred times better resolution. Sensitivity refers to the ability of an eye to get enough light to the receptor cells, whereas resolution is the precision with which light rays from different directions are kept separate (to prevent blurring).
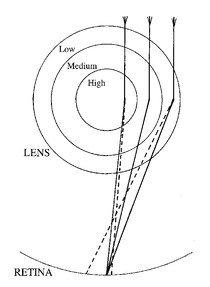
Figure 7.3
Focusing of light rays (solid lines) by a graded lens (only three grades of material are shown). The dashed lines represent the paths induced by a standard lens of uniform material - the steeper angles of contact cause light to be bent more at the periphery. The core material of the graded lens, however, causes light to bend more than the material of the periphery layer, and so counteracts this angular discrepancy.
In eyes of vertebrates on land, between 20 and 67 per cent of the focusing power is supplied by the cornea. So the lens can be designed specifically to correct blurring and to make accommodation for different distances - nearby objects would otherwise be imaged further away from the lens than distant ones. Mammals, birds and most reptiles meet these goals by changing the shape of their lens or cornea, thereby adjusting the position of the focal plane. They use tiny muscles to pull and stretch the lens. Alternatively, fish, frogs and snakes move their lenses backwards and forwards. Lens movement can provide adjustments for water or air in some amphibious animals.
Although most spiders are equipped only with ocelli, jumping spiders and wolf spiders are exceptions. They have camera-type eyes with a thick cornea, which provides all the focusing power. The principal eyes of the jumping spider are unusual because, like birds of prey, they have a large pit in each retina that acts as a negative lens - it inverts and magnifies the image. This is comparable to the rear element of a telephoto lens in a camera.
The principal eyes of the jumping spider are also unusual because their retinas are narrow vertical strips, giving a restricted visual field of only a few degrees in the horizontal direction but about 20° in the vertical. These retinas move sideways to compensate for their thinness, and so greatly extend the field of view in the manner of a photocopier scanning a picture. A similar mechanism is found in some snails and crustaceans. In some other crustaceans, such as the iridescent
Sapphirina
with the appearance of a swimming opal, there is a more extreme development. Here the retina is merely a dot with only a few light detection cells, but constant movement in all directions puts these cells to continual use.
The iris controls the pupil size and, consequently, the amount of light entering the eye, in the manner of a camera diaphragm. But a further
mechanism for brightness control may also exist in camera-type eyes, involving a reflector lying behind the retina. Like the scallop eye, the eyes of some vertebrates also contain a mirror behind their retinas, again employing the silver fish-mirror mechanism. But here the mirror functions so as not to focus the light - light is prefocused by a lens. In this case, the mirror provides an adaptation to the night. In the dark-adapted state, the reflector returns to the retina light rays that initially passed between the retinal cells without detection. So the most is made of the light available - anything not detected first time will have a second chance. The reflector in the eyes of cats and crocodiles reflects the beams of headlights and torches, often appearing obvious as âeyeshine' at night. This represents the light missed both first
and
second time by the retina. When light levels are very low,
all
the rays striking the eye become invaluable to vision - the visual frontier between sight and blindness is approached. But when light levels are high, the reflector is redundant and becomes covered by a dark absorbing pigment. This mechanism is actually common in many nocturnal animals with camera-type eyes.
Briefly stepping back over the visual threshold, into ânot to see' territory, some light perceivers actually contain a retina and a lens. Included among these are the receptors of scorpions, many web-building spiders and most snails. But their small size is important. This receptor type is not an âeye' because it cannot focus an image on its retina - the retina is simply too close to the lens. The likely function of this receptor is to measure the average brightness or colour over large angles. As mentioned already, we are not so concerned with these light perceivers in this book, but they do help to illustrate just how much information can be acquired just from the architecture and size of a structure containing a lens and retina. The reason for writing about today's eyes in this chapter is merely to provide a palaeontological tool. Where internal views of a simple eye-like structure are possible, we can calculate whether visual images were formed - whether it really was a simple
eye
. And the
size
information will become even more important when we consider fossils in relation to the Cambrian enigma. But fossil eyes that reveal internal architecture are extremely rare. So the best studied fossil eyes belong to the second type of eye found in
animals, one where more information on sight can be deduced from just an outer surface. At least half the animals on Earth today are equipped with compound eyes.